I’ve recently completed my secondment at Artinis Medical Systems BV, where the main purpose was to familiarize with functional near infrared spectroscopy (fNIRS). This is an imaging technique that can help us study regions of the brain that activate with incoming stimuli. In our case, we’re interested in auditory stimulation. Two things were really important for me during this process. Firstly, due to some relaxations in Covid measures, my secondment became “in-person”, which meant I was able to be physically present at the office, meet new people and play around, in a hands-on setup, with their devices. Secondly, I was able to learn basic aspects about fNIRS and even perform a couple of preliminary measurements.
First, let’s talk about my hosts. Artinis is an innovative Dutch company that has been providing for almost two decades solutions for fNIRS measurements and radiological quality management. Their headquarters are located in Elst, The Netherlands. This was already a great start, since it meant cycling distance from my current home. People at Artinis were really nice and welcoming. Furthermore, being manufacturers, they have solid expertise in NIRS technology. With their guidance, I learnt about the basics, completing a general overview of fNIRS, how their devices work, connections and synchronization with other systems, such as EEG devices (see Loes’ adventures for a nice description of EEG), plus some signal processing, among other things.
But, what is fNIRS? As stated earlier, this stands for functional near infrared spectroscopy. Long statement, so let’s go by parts. The term functional comes from functional neuroimaging, which is the use of neuroimaging technology to measure brain functions. Spectroscopy is the study of interactions between matter and electromagnetic radiation. In this case, this electromagnetic radiation is near-infrared light. Putting everything together, fNIRS is a neuroimaging technique that uses light to measure brain activity.
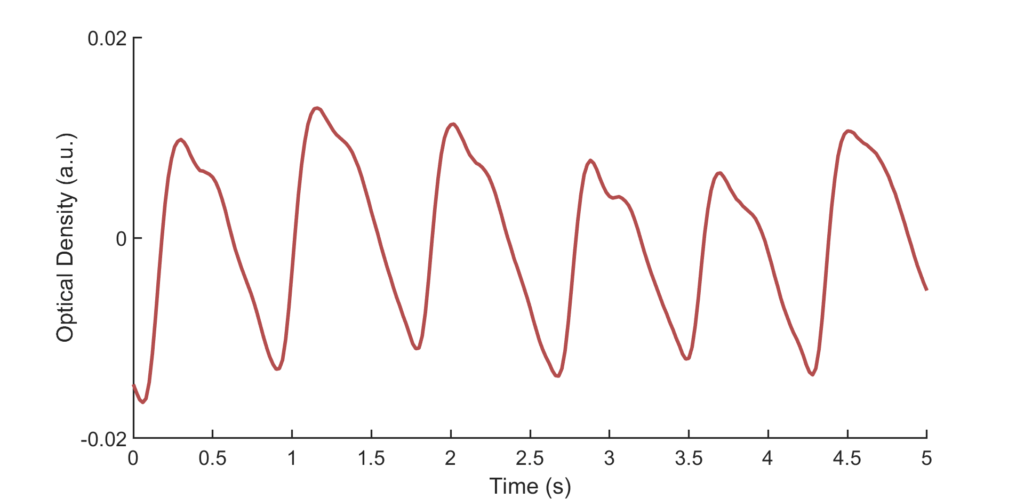
I’m currently focusing on using this technique to evaluate how the brain detects and discriminates different sounds. The end goal is to search for differences in brain activation between cochlear implant recipients who perform poorly and those who have excellent speech recognition abilities. First, we are testing normal hearing participants, then, we’ll try to extend our methods to cochlear implant recipients.
How does this work in practice? We first place transmitters and receivers on the head of participants. This involves adjusting a cap similar to those used in EEG. Through a harmless and non-invasive process, the transmitters shine near-infrared light through the participant’s head and the receivers measure the amount of light that comes back. On the path from the transmitter to a receiver, some light is absorbed. This includes absorption by haemoglobin, the protein that makes our blood look red and that is in charge of transporting oxygen through our bloodstream. When neurons in our brain become active, for example, due to the incoming sounds, they demand resources to keep up their activity. One of these resources is oxygen, which they do not store by themselves. We can use fNIRS signals to detect changes in oxygenated and deoxygenated haemoglobin. Regions that activate due to the sound stimuli will tend to exhibit an increase in oxygenated and decrease in deoxygenated haemoglobin, thus marking activation.
What’s attractive about this technique is that devices can be made lightweight, portable and rather easy to set up. Besides, the optical nature of this technique implies that cochlear implant users are not exposed to strong magnetic fields, as would be the case for functional magnetic resonance imaging (fMRI), another imaging technique commonly used to investigate the brain’s response to auditory stimuli. In fMRI, the internal magnet of a cochlear implant represents a challenge, due to the safety limits it imposes on the magnetic field and imaging artefacts.
Can we use fNIRS to shed some light on cochlear implant users’ performance? We’ll have to find out!
What’s next? At Artinis, I completed a series of preliminary measurements which I’m looking forward to continue at the Biophysics laboratories of the Radboud University in Nijmegen. First, we need to complete the set-up at the lab, test the equipment and perform some initial measurements. Then we can launch a larger study, starting with normal hearing participants, then moving to cochlear implant recipients. Can we use fNIRS to shed some light on cochlear implant users’ performance? We’ll have to find out!